Versatile sandwich panel technology solves decades-long exterior automotive challenge. #focusondesign
RLE International developed an innovative, low-cost/low-pressure compression molding variant and thermoplastic sandwich panel technology that has been in production on the interior bulkhead (above) of a commercial vehicle since 2019. The technology is now being investigated to produce exterior roof panels and hoods. Photo Credit: SABIC microfiber leather
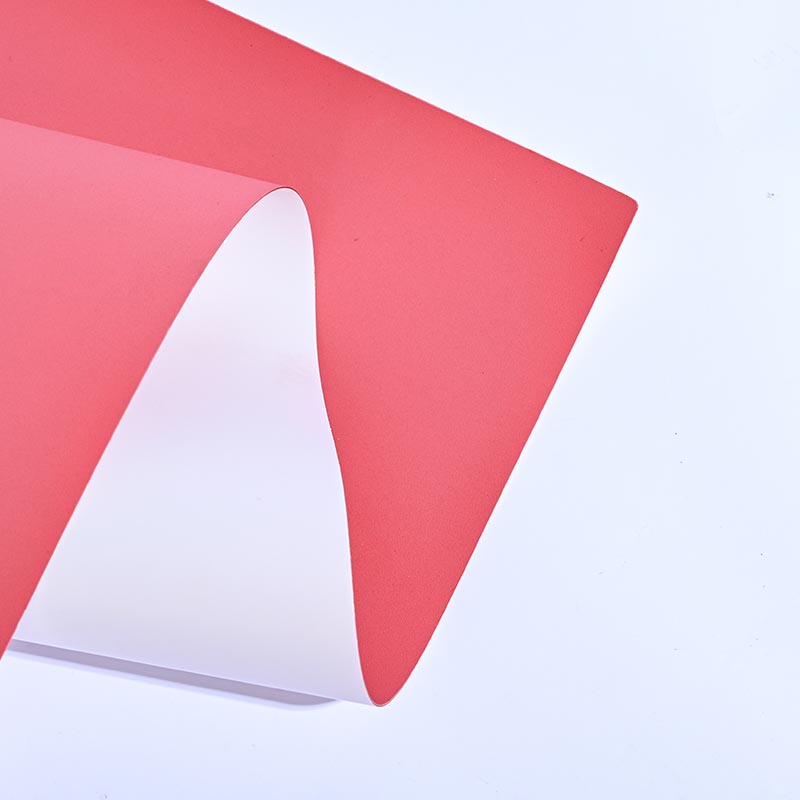
For at least five decades, significant work across the global automotive composites supply chain has focused on trying to make thermoplastic composites a viable option for horizontal body panels with a Class A finish, including hoods, roofs and decklids (trunk lids). (Below, sidebar A provides a short history of composite body panels and sidebar B discusses why this is desirable.) Thermoset composites replaced metals on vehicle exteriors in semi-structural/vertical and fully structural/horizontal body panels starting in the 1950s. Thermoplastic composites have been used intermittently on vertical body panels like bumper fascia and fenders since the 1980s, door panels and other trim since the 1990s and liftgates since the early 2000s. However, it has proven difficult to meet the auto industry’s performance, cost and aesthetic requirements in horizontal panels with this class of materials.
That may be changing thanks to a low-pressure variant on compression molding and thermoplastic sandwich panel technology out of the U.K. For four years, the technology has been in production on a large bulkhead for a light commercial vehicle rated to carry 2 tons/tonnes of cargo. More recent work, which is discussed here, suggests the technology may offer the right combination of design flexibility, mechanical performance, affordable and rapid processing and surface aesthetics to be viable on horizontal exterior panels at last.
Eight generations of the composite-bodied Chevrolet Corvette sports car from General Motors Co. highlights an interesting progression of composite materials used for body panels. Photo Credit: General Motors Co.
Thermoset composites have a long history of use on passenger vehicles for semi-structural vertical and fully structural horizontal exterior body panels as well as elements of the chassis/monocoque. Glass fiber-reinforced plastic (GFRP) debuted in 1953 on all exterior panels of the new Chevrolet Corvette sports car from then General Motors Corp. (now General Motors Co., GM, Detroit, Mich., U.S.). In the 1960s, hand layup FRP was replaced with faster cycling and more consistent compression molded sheet molding compound (SMC) on the Corvette Since then, SMC’s use has expanded beyond body panels to encompass structural pickup boxes, chassis components and, more recently, large protective enclosures for electric vehicle battery packs (read CW’s Part 1 and Part 2 articles on this application). Additionally, carbon fiber/thermoset matrix composites transitioned from significant portions of Formula 1 and 2 race cars in the 1990s to body panels and later entire monocoques of street-legal cars (including the Corvette) starting in the early 2000s.
Injection molded short glass fiber-reinforced thermoplastics debuted on non-structural bumper fascia in the early 1980s and by the mid-1980s were transitioning to other vertical panels like fenders. In 1990, when GM’s Saturn cars debuted, all vertical body panels were injection molded thermoplastic composite. However, the hood, decklid and roof stayed in stamped steel and eventually the entire exterior body transitioned to steel before production ended in 2010.
Several other GM nameplates sported thermoplastic vertical panels that decade, as did vehicles like the limited-edition (1989-1991) Z1 roadster from BMW AG (Munich, Germany) and later the diminutive smart fortwo micro car that then Mercedes-Benz division of Daimler AG (now Mercedes-Benz AG, Stuttgart, Germany) launched in 1997. smart was the first car to feature all thermoplastic body panels, although hood and roof panels were very small indeed. In 2007, smart became the first passenger car to switch from fiberglass-reinforced polycarbonate/polybutylene terephthalate (GR/PC-PBT) to talc-filled thermoplastic polyolefin (TPO).
Over the last 15 years, talc-filled TPOs have slowly displaced engineering thermoplastics like GR/PC-PBT and GR/modified-polyphenylene ether-polyamide (GR/MPPE-PA) on vertical body panels. Another significant milestone that was 10 years in the making occurred in 2014 with the debut of an all-thermoplastic liftgate (a talc/TPO outer panel bonded to a long fiber thermoplastic polypropylene (LFT-PP) inner panel) on Nissan Rogue cross-over utility vehicles (CUVs). Since then, other SUVs and CUVs have followed suit.
The story begins circa 2015 when an automaker’s engineering team approached the U.K. offices of RLE International (Cologne, Germany) for help taking cost and mass out of a commercial vehicle. RLE is internationally known for its automotive design and engineering work, particularly on primary body structures and interiors. As such, the company provides full CAE, engineering, styling feasibility and launch support. RLE’s team proposed several metal-to-composite conversion options to reduce cost and mass, including fully structural/horizontal exterior roof panels and a semi-structural/vertical interior bulkhead between cabin and cargo hold.
The joint team tackled the technically easier bulkhead first. Over the next few years, design of the bulkhead evolved such that the technology eventually passed all OEM performance requirements. To meet the OEM’s maximum 12-kilonewton impact requirement, the final bulkhead features skins of a single layer of lightweight reinforced thermoplastic (LWRT)— comprising a polypropylene (PP)-impregnated loftable, short glass, low grams/square-meter (GSM) form of glass mat thermoplastic (GMT) composite material — sandwiching a core of 0°/90° unidirectional fiberglass tapes (although fabric weaves also could be used). The LWRT skin materials were selected to provide a good unpainted surface finish.
Bulkheads are molded in <90 seconds via a low cost/low pressure (3.4-4.5 bar) “thermopressing/consolidation” variant on compression molding in matched metal dies at room temperature. The tool is neither heated nor chilled, but materials used in the sandwich structure are preheated before forming. Since the tool doesn’t have a shear edge, waterjet trimming is required after demolding to clean up the part edge and cut any required holes. The one-piece panel replaced a multipiece steel/polyurethane foam liner assembly, reduced mass by 5.2 kilograms, lowered piece cost and decreased tooling investment by 80%. Additionally, the all-fiberglass/olefin sandwich structure is fully recyclable at end of part life. Commercial since 2019, the award-winning bulkhead is produced in volumes of 60,000-70,000/year, although reportedly the process can produce up to 90,000 parts/year from a single tool.
Believing that the same technology would work for an external roof panel, RLE’s team — led by Mark Grix, head of interior/exterior development — opted to move forward on its own to develop a Class A-capable horizontal roof panel. Grix has developed thermoplastic exterior body panels since his days at GE Plastics in the 1990s.
Since the team had a modest budget, clever approaches were needed. One such approach involved obtaining three free exterior steel roof panels — intended for use as the outer/skin layer of welded roof panels on the same commercial vehicle that sports the bulkhead. The panels did double duty. One was scanned and used to create a template to rapidly design the panel’s outer skin, significantly reducing CAE time. Since the interior (B side) of the roof panel wasn’t part of the proof of concept, the team focused solely on exterior (A-side) geometry and surface finish.
The other two steel panels were used to create a low-cost forming tool, with one skin functioning as the core and the other as the cavity to mold the A and B surfaces of the relatively flat composite roof panel. The roof tool was subsequently used to form composite panels during the development program and Grix says it produced excellent parts right from the start.
Beginning with a sandwich structure similar to that used for the commercial bulkhead, a number of different combinations of skins and cores (in total thicknesses ranging from 3 to 5 millimeters) were laid up, molded and evaluated to develop the roof panel concept. Once the various materials are preheated (different layers may be subjected to heat for different lengths of time), they are manually laid up in a compression press loaded with the aforementioned tool (held at room temperature) and formed via thermopressing. Researchers again targeted an effective cycle time of 90 seconds.
Working without OEM specs for the developmental roof panel, RLE focused its efforts on the exterior skin, with the intention of keeping roof hoops/bows in place and bonding the composite solution to an existing weld flange used for the current metallic roof. This was possible because the composite structure was formulated to have a low coefficient of linear thermal expansion (CLTE). Subsequent testing has shown the target thermoplastic panel has a CLTE close to that of aluminum. Photo Credit: RLE International
Roof panels were molded at a U.K. facility owned by Airborne (The Hague, Netherlands) in order to further develop the concept and achieve the right combination of skins and cores for the sandwich panel construction. Airborne’s facility was ideal, since it was equipped with a very large oven capable of heating the entire 1.75 × 2.0-meter roof panel and could heat materials to 240°C. Ironically, the oven actually proved too large for the panel and work was done to section off part of the oven so material didn’t have too long a dwell time, and to permit material to be fed in from the side partway down the length of the oven rather than from the end.
Various combinations of panel materials were subsequently subjected to small-scale lab tests. Eventually, the most promising candidate was bonded — via 1K structural urethane adhesive from Sika AG (Baar, Switzerland) — onto the roof hoops/bows of a test vehicle and subjected to additional vehicle-level testing.
Since the final composite is assembled in the press just before consolidation and forming, depending on the desired properties and surface finish required for an application, RLE’s technology offers great versatility regarding the types of materials that can be combined to form the final panel’s skins, cores and exterior surface finish. Nearly any type of sheet-form thermoplastic composite can be used (e.g., tapes, GMT, organosheet, LWRT). The only requirements are that polymer matrices be chemically compatible (to achieve good material flow, ensuring a homogeneous component), and have similar glass transition temperatures (Tg) to permit heating in the same oven without worrying that higher-temp material will melt lower-temp ones before consolidation/forming is complete.
“Every application’s requirements are going to be different, so we have the flexibility to work with materials with FVFs [fiber volume fractions] ranging from a high 20% to the 50% range, although we typically like to come in around 30-40%,” explains Grix. “Testing has shown us there’s no benefit to be gained by using higher FVFs. And, in fact, if we have too much glass [to resin], then impact strength and adhesion between layers suffers. To get a good surface, it is absolutely essential to have enough resin.”
For the top-most aesthetic layer, which controls surface finish, candidates include matte or gloss thermoplastic films, textiles, scrim/fabric combinations or even scratch/UV-resistant hardcoats or soft-touch thermoplastic polyurethane (TPU) coatings. Again, the beauty of the technology is that it can easily be modified on-the-fly to meet application needs in many industries.
To date, RLE has produced automotive Class A-capable panels in durable and UV-resistant gloss or matte surfaces directly from the mold using precolored films and low-cost aluminum tooling in a cycle time of <2 minutes. Compared to steel panels, composites offer a potential mass savings of 50% for structural panels and 60% for exterior/semi-structural panels. They also offer excellent impact strength even at low temperatures and full end-of-life recyclability. This thermoplastic sandwich panel technology is gaining interest among not just automotive industry players but also those in other ground transportation segments. Photo Credit: RLE International
The team looked at different combinations of thermoplastic resin systems (primarily in the olefin and polyamide families) and reinforcements (including chopped, unidirectional and woven fabrics) for the skins and cores of the roof’s sandwich panel. Owing to its elevated position, the developmental roof panel didn’t require a Class A finish. Initially, precolored black material was used and matte white panels were also tried. More recently, Grix and his team have improved UV stability and scratch resistance by applying a film layer in the tool as well as trying a hardcoat finish. The panel can use a contrast color or it can match the rest of the body. No metallic reinforcements are required in either the developmental roof panel or the commercial bulkhead. Should the entire steel roof — not just a single panel — be converted to the thermoplastic sandwich technology, Grix estimates a mass savings of 24.5 kilograms could be achieved.
A big question automakers like to ask is what technology readiness level (TRL) a concept currently is at, and Grix’s answer is that the roof panel concept is fairly far along.
“You’ll still need to do some OEM-specific testing, like environmental stability, NVH [noise/vibration/harshness], and things like that, but for all intents and purposes the roof panel uses the same material family and a variation of the same processing method as the bulkhead, which has been in fairly high-volume commercial production for the last four years,” he explains. “The flexibility of this technology means that if you need to increase UV performance or scratch resistance, or even to make the panel thicker or thinner or more rigid, then we can do that. We’ve spent a lot of time really working to understand this technology and what’s required to make nice panels right out of the tool.”
Another developmental project that RLE has investigated is using the thermopressing/sandwich panel technology to produce a hood. In this case, the outer panel used a higher temperature polyphthalamide (PPA) matrix. Again, the team found mass savings greater than 50% versus steel and excellent PedPro performance in a recyclable panel. Photo Credit: RLE International
RLE reportedly is in discussions with automotive Tier 1s and OEMs in other ground transportation segments like farm equipment and golf carts to commercialize both interior and exterior applications.
What’s next for this technology? “There’s no reason (with the right formulation) this technology can’t be used for PedPro-compliant [pedestrian protection-compliant] hoods too,” Grix says. “We’ve already produced a developmental hood, and small-scale testing shows we have better energy absorption and dissipation than thermosets or steel. Also, we’ve explored using this technology not just for relatively simple panels but for high-draw panels for vehicle interiors with significantly more geometric complexity. Combine that with a Class A precolored finish right out of the tool, mass savings of 50-60% versus steel and a fully recyclable part at end of life and we feel this is a winning combination for OEMs looking for lightweight panel technologies in multiple industries. We’d love to work with anyone interested in exploring this further.”
Compared to either thermoset composites or metals, thermoplastic composites are desirable for exterior body panels for many reasons. First, they tend to be lighter (lower specific gravity), they have better damage tolerance (impact strength) — a bonus for meeting PedPro requirements — and they provide better surfaces out of the tool, reducing post-mold finishing.
Unlike thermosets, thermoplastic composites are recyclable (melt reprocessable), making it easier to reuse scrap and recover usable material from end-of-life parts — an important feature for those selling vehicles into the EU — and they’re also supplied fully polymerized, meaning molding cycles tend to be shorter and offer better repeatability and reproducibility (R&R), which makes them better suited for higher volume programs and helps offset their typically higher raw material and tooling costs. They also offer greater design freedom and ample opportunities for parts integration (to reduce part count), insertion of hardware (to facilitate joining) and simplified post-mold assembly versus metals.
Despite all their benefits, thermoplastic composites — like all materials — have challenges that must be overcome. For example, owing to higher CLTEs than steel or aluminum, early monolithic thermoplastic composite body panels with discontinuous fiber reinforcement required that larger-than-desirable gaps be left between composite and metallic panels to accommodate dimensional changes during temperature cycling. Without this aesthetic tradeoff, moveable panels like doors couldn’t be opened or closed at times. That has largely been overcome by switching from monolithic panels to pairs of bonded inner/outer panels — with or without cores — and by using longer chopped/discontinuous fiber reinforcement as well as continuous fiber nonwoven, unidirectional and woven reinforcements in sheet form composites like GMT/organosheet and thermoplastic tapes.
Another problem was that the thermoplastic grades the automotive industry favors — largely PP, PA 6 or 6/6 and blends of PA or thermoplastic polyester with PC or MPPE — lacked thermal stability to endure bake oven temperatures after electrophoretic coating (e-coat) of the body-in-white (BIW) and painting. That added assembly complexity and cost, since panels had to be painted offline and added at a later stage in the vehicle assembly process. Although painting of thermoplastic panels is still accomplished offline, much work has been done to improve the UV stability, depth-of-color and metallic special effects of molded-in-color (MIC) panels, which then only require a clear coat to finish. Additionally, great strides have been made in film technology, which offers more durable surfaces than paint and aligns with automaker efforts to reduce the cost and environmental burden of painting.
Still another formulating problem was striking the right balance between stiffness and impact to avoid the need for large metallic brackets on which vertical panels hang from the vehicle. A related issue is that since stiffness and strength are often lower than values for metals, geometry (such as ribbing) was often needed to achieve comparable mechanical performance in panels. That, however, tended to lead to thicker nominal walls, which can cause packaging issues. Faced with the prospect of having to redesign body structures on existing vehicles to accommodate thicker composite panels, most OEMs will just pass. For TPO materials, careful formulation work that combines both discontinuous fiber reinforcement and mineral fillers has led to these materials dominating skins on exterior vertical panels. Also, by switching from a monolithic panel design to a bonded inner/outer panel, a structural inner panel that wouldn’t meet Class A requirements is complemented by a Class A-capable outer skin that wouldn’t meet mechanical requirements alone.
Perhaps the greatest challenge that made thermoplastic horizontal panels unviable for decades was the issue of sagging and long-term creep at elevated temperatures. There were several reasons for this. First, thermoplastics soften over a range of temperatures close to their Tg, which causes a progressive loss of mechanicals including the ability to carry loads and be self-supporting. The property that makes them easier to recycle can also compromise their long-term mechanical integrity at elevated temperatures. To an extent, that is helped by switching from short/discontinuous fiber reinforcement to continuous fiber options, and moving from monolithic panels to bonded panels with or without cores.
A related issue is how to achieve sufficiently high FVFs or FWFs to improve mechanicals during periods of elevated temperatures. The fact that thermoplastic polymers are supplied prepolymerized (with very long molecular chains before molding) means that even at melt temperatures, they are fairly viscous compared with many liquid thermoset systems. That makes it hard to achieve high levels of fiber wetout and impregnation. Hence, even today, there are limited injection molding grades with FWFs above 40%, whereas many thermoset technologies permit FVFs as high as 70% to be achieved. To an extent, this has been addressed by a combination of careful resin selection (e.g., using branched versus linear forms of PP with lower viscosity), modifications to the lamination process for thermoplastic tape and GMT/organosheet production and, more recently, impregnation with low-viscosity caprolactam monomer that is subsequently reactively polymerized into PA6. The tradeoff is longer cycle times (more like thermosets) and specialized equipment. However, the increasing ability over time to get more fiber, particularly more long and continuous fiber, into thermoplastic composites has been a great enabler for their use in increasingly structural applications. The one tradeoff here is that for exterior aesthetic surfaces, higher FVFs/FWFs can lead to fiber readthrough. This can be addressed, in part, by using films, by painting or texturing the panels or by using lower fiber reinforcement in the outermost aesthetic layers in sandwich panel compositions.
Last but not least, direct and indirect costs are always a sensitive point in the high-volume/low-margin automotive industry. While the composites industry was working on material and process advancements to address one body panel challenge after another, so too did counterparts in the aluminum and steel industries improve their materials and processes. Even today, unless switching to composites permits significant mass to be removed from a vehicle, provides important manufacturing benefits or enables new levels of safety or functionality to be added, most automakers will balk at using composites unless the cost to do so is at least neutral or lower than that of conventional materials.
Voith Composites and partners develop recycling solutions for hydrogen storage tanks and manufacturing methods to produce automotive parts from the recycled materials.
HyPatchRepair project proves feasibility of automated process chain for welded thermoplastic composite patch repairs.
Automotive and filament winding experts at Voith Composites showcased their Type IV hydrogen storage tanks at JEC 2023. These 350-liter pressure vessels, manufactured with recycled carbon fiber, are primarily designed for heavy-duty vehicles.
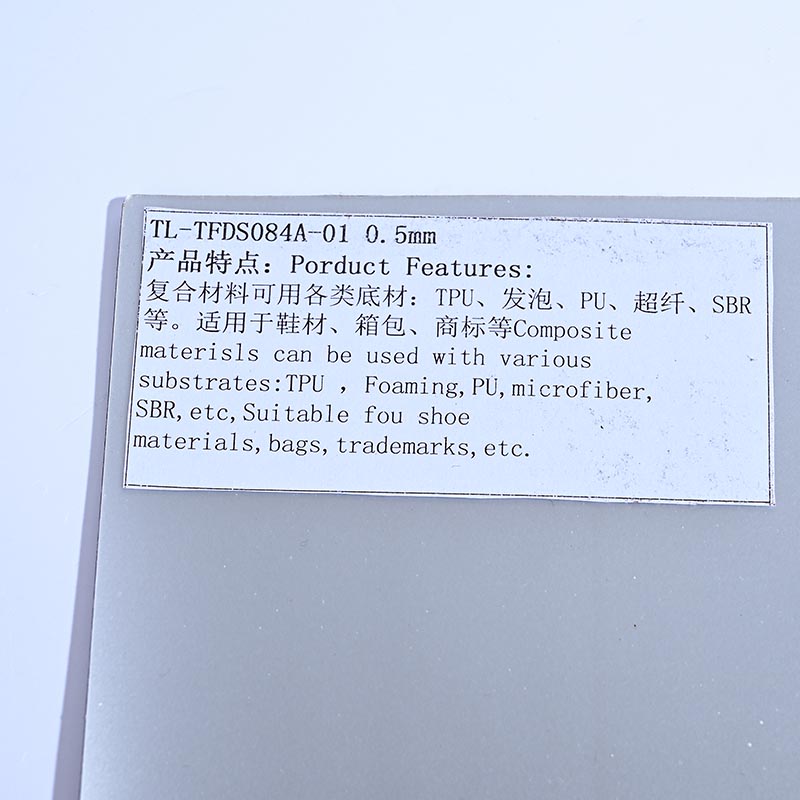
Composited Tpu Material © 2023 Gardner Business Media, Inc. Privacy Policy [Log On]